Exotic New Superconductors Delight and Confound
Introduction
This year, superconductivity — the flow of electric current with zero resistance — was discovered in three distinct materials. Two instances stretch the textbook understanding of the phenomenon. The third shreds it completely. “It’s an extremely unusual form of superconductivity that a lot of people would have said is not possible,” said Ashvin Vishwanath (opens a new tab), a physicist at Harvard University who was not involved in the discoveries.
Ever since 1911, when the Dutch scientist Heike Kamerlingh Onnes first saw electrical resistance vanish, superconductivity has captivated physicists. There’s the pure mystery of how it happens: The phenomenon requires electrons, which carry electrical current, to pair up. Electrons repel each other, so how can they be united?
Then there’s the technological promise: Already, superconductivity has enabled the development of MRI machines and powerful particle colliders. If physicists could fully understand how and when the phenomenon arises, perhaps they could engineer a wire that superconducts electricity under everyday conditions rather than exclusively at low temperatures, as is currently the case. World-altering technologies — lossless power grids, magnetically levitating vehicles — might follow.
The recent spate of discoveries has both compounded the mystery of superconductivity and heightened the optimism. “It seems to be, in materials, that superconductivity is everywhere,” said Matthew Yankowitz (opens a new tab), a physicist at the University of Washington.
The discoveries stem from a recent revolution in materials science: All three new instances of superconductivity arise in devices assembled from flat sheets of atoms. These materials display unprecedented flexibility; at the touch of a button, physicists can switch them between conducting, insulating, and more exotic behaviors — a modern form of alchemy that has supercharged the hunt for superconductivity.
It now seems increasingly likely that diverse causes can give rise to the phenomenon. Just as birds, bees and dragonflies all fly using different wing structures, materials seem to pair electrons together in different ways. Even as researchers debate exactly what’s happening in the various two-dimensional materials in question, they anticipate that the growing zoo of superconductors will help them achieve a more universal view of the alluring phenomenon.
Pairing Electrons
The case of Kamerlingh Onnes’ observations (and superconductivity seen in other extremely cold metals) was finally cracked in 1957. John Bardeen, Leon Cooper and John Robert Schrieffer figured out (opens a new tab) that at low temperatures, a material’s jittery atomic lattice quiets down, so more delicate effects come through. Electrons gently tug on protons in the lattice, drawing them inward to create an excess of positive charge. That deformation, known as a phonon, can then draw in a second electron, forming a “Cooper pair.” Cooper pairs can all come together into a coherent quantum entity in a way that lone elections can’t. The resulting quantum soup slips frictionlessly in between the material’s atoms, which normally impede electric flow.
Bardeen, Cooper and Schrieffer’s theory of phonon-based superconductivity earned them the physics Nobel Prize in 1972. But it turned out not to be the whole story. In the 1980s, physicists found that copper-filled crystals called cuprates could superconduct at higher temperatures, where atomic jiggles wash out phonons. Other similar examples followed.
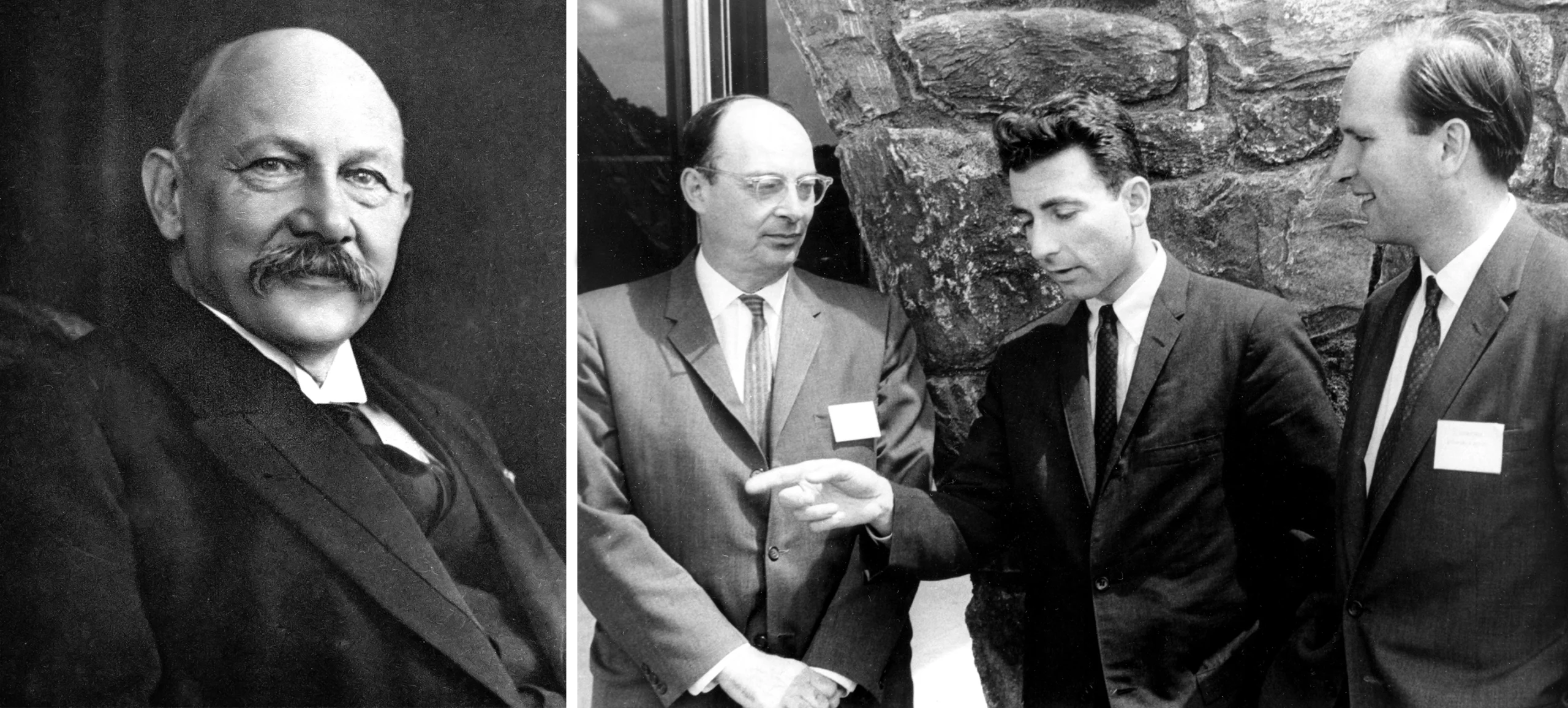
Heike Kamerlingh Onnes (left) stumbled upon superconductivity in 1911. An explanation for it eluded Albert Einstein and other luminaries until the 1950s, when John Bardeen, Leon Cooper and John Robert Schrieffer (right photo, left to right) determined that atomic vibrations known as phonons were at work.
From left: Chronicle/Alamy; AIP Emilio Segrè Visual Archives
Theorists brainstormed new ways of pairing electrons.
The higher-temperature superconductors seemed to have atoms arranged in a way that slows electrons down. And when electrons get the chance to mingle in a leisurely fashion, they collectively generate an ornate electric field that can make them do novel things, like form pairs rather than repel. Physicists now suspect that in cuprates, specifically, electrons hop between atoms in a particular way that favors pairing. But other “unconventional” superconductors are still quite mysterious.
Then, in 2018, a new superconductor opened physicists’ eyes wider.
Pablo Jarillo-Herrero (opens a new tab), a physicist at the Massachusetts Institute of Technology, found that if you took a sheet of carbon atoms arranged in a honeycomb lattice — a 2D crystal called graphene — twisted it at precisely 1.1 degrees, and stacked it on top of another graphene sheet, the two layers could superconduct.
Researchers had already been dabbling with 2D materials and finding diverse behaviors. By applying electric fields, they could add electrons to the sheet or make the electrons feel almost as if the atomic grid were contracting. Twiddling these settings in a single 2D device could reproduce the behavior of thousands to millions of potential materials. Among those heaps of possibilities, Jarillo-Herrero had shown, was a new superconductor: “magic angle” graphene.
Then, a couple of years later, a group in California removed the magic angle, finding that three-layer, twist-free graphene devices could also superconduct.
Mark Belan/Quanta Magazine
Researchers are still discussing why electrons stick together in these cases. Phonons fit the data in some ways, but something new also seems responsible.
But what really thrilled physicists was the promise of a fresh way to investigate superconductivity in general. The customizable 2D devices had freed them from the drudgery of designing, growing and testing new crystals one by one. Researchers would now be able to quickly re-create the effects of many different atomic lattices in a single device and find out exactly what electrons are capable of.
The research strategy is now paying off. This year, physicists found the first instances of superconductivity in 2D materials other than graphene, along with a completely novel form of superconductivity in a new graphene system. The discoveries have established that the earlier graphene superconductors mark just the outskirts of a wild new jungle.
A Hint Vindicated
In 2020, the physicist Cory Dean (opens a new tab) and his team at Columbia University tried stacking sheets of a different 2D crystal — this one, a honeycomb arrangement of two types of atoms, called a transition metal dichalcogenide (TMD). When they twisted the sheets at 5 degrees, the resistance plunged toward zero (opens a new tab) but didn’t stay there. It was an inconclusive hint of superconductivity.
The tentative nature of the detection didn’t stop Liang Fu (opens a new tab) of MIT and Constantin Schrade of Louisiana State University from trying to explain it (opens a new tab). They suspected that phonons weren’t the answer. Twisted materials are powerful because the twist changes what the electrons experience, imbuing the material with a kaleidoscopic “moiré” pattern. The moiré features large hexagonal cells that act like artificial atoms, hosting electrons. In this new environment, electrons move slowly enough for their collective electrical interactions to guide their behavior.
5W Infographics; Mark Belan/Quanta Magazine
But how were the electrons conspiring to form pairs? The Columbia group funneled electrons into the moiré. They observed that when there was one electron for each of the large cells in the moiré material, these electrons assumed an “antiferromagnetic” arrangement; their intrinsic magnetic fields tended to alternate between pointing up and down. Adding extra electrons to the moiré made the resistance drop to zero — Cooper pairs had formed. Fu and Schrade argued that the same electron-on-electron action was making both the antiferromagnetic state and the superconducting state possible. At one electron per cell, each electron can have a preferred location and magnetic orientation. But when additional electrons pile in, the magnetic arrangement becomes unstable, and the whole population starts to flow freely.
Scientific journals initially rejected Fu and Schrade’s paper describing these ideas because there wasn’t any hard evidence that TMDs can superconduct. Now there is. The Columbia group spent the last four years improving their ability to measure electrical resistance at low temperatures, and earlier this year they had a breakthrough. They assembled another two-sheet device with a 5-degree twist, cooled it down, and watched it superconduct (opens a new tab) — an observation soon to be published in Nature. “Lo and behold, we see that the state that we thought existed is appearing in exactly the right place,” Dean said. “It’s a little bit of vindication.”
Fu and Schrade’s theory — bolstered by the Columbia confirmation — has now been published, but it isn’t proved. One way to test it is to check whether the Cooper pairs can rotate, as the theory predicts. That’s an unusual feature, as electrons paired by phonons don’t orbit each other.
Adding electrons to an antiferromagnetic metal isn’t the only way to cook up superconductivity in TMDs. Shortly before the Columbia discovery, another group found an even more peculiar species of superconductivity in the very same material.
Jie Shan (opens a new tab) and Kin Fai Mak (opens a new tab), an academic power couple who run a lab at Cornell University, had been searching for superconductivity in TMDs since Jarillo-Herrero’s blockbuster twisted-graphene discovery in 2018. They spent years mixing and matching five kinds of TMD crystals, trying out different twist angles and temperatures, and applying various electric-field strengths to the material — searching a massive haystack for a superconducting device.
When the needle finally appeared, it displayed a species of superconductivity that no one had seen coming. The Columbia team had started with an antiferromagnetic metal and added electrons. The Cornell group, however, started with an insulator and added nothing. Their moiré pattern — which resulted from a milder 3.5-degree twist — allowed electrons to slow down so much and interact so strongly that they all got stuck in place at precisely one electron per cell.
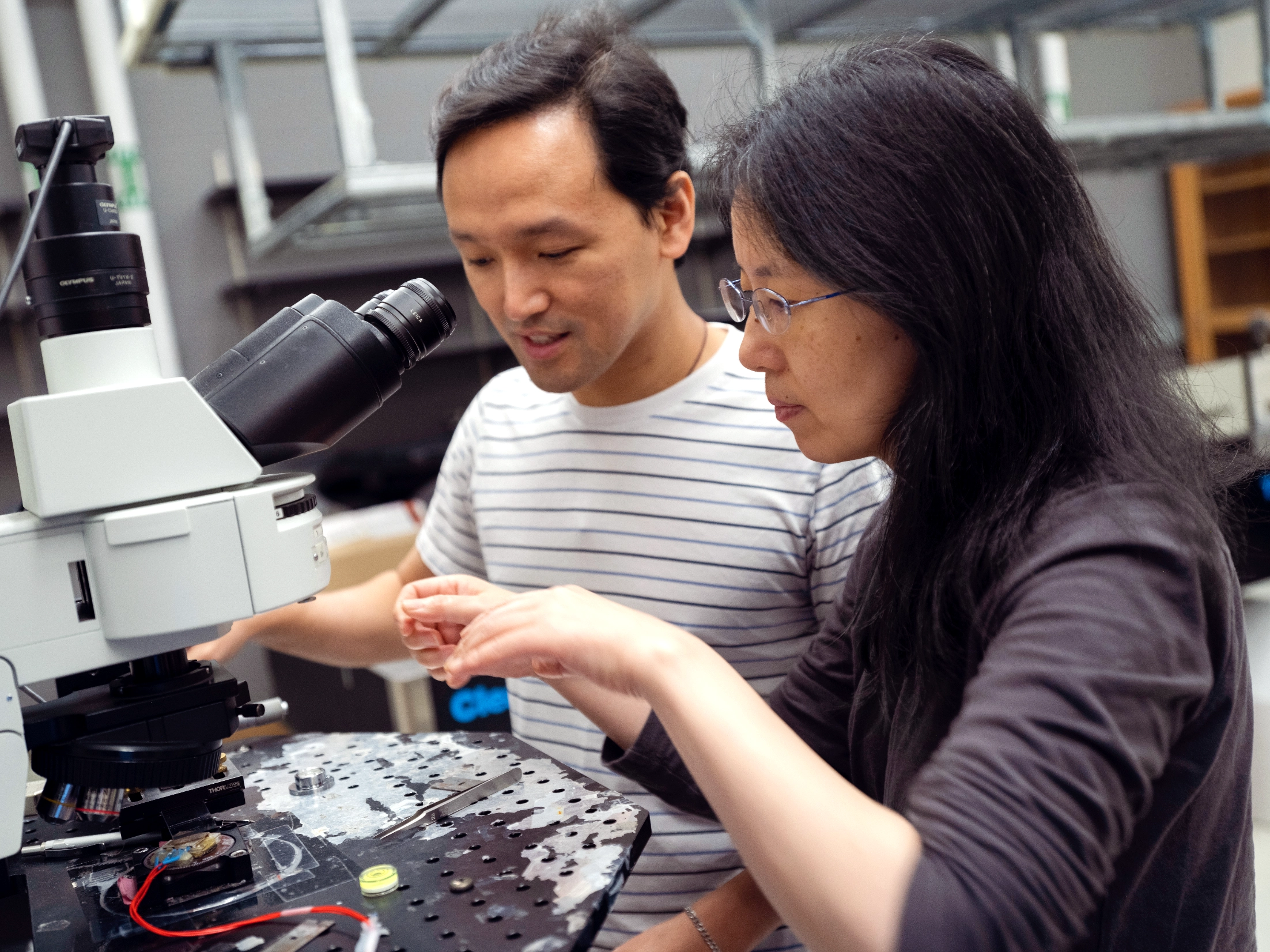
A team at Cornell University led by Kin Fai Mak (left) and Jie Shan recently discovered an unusual sort of superconductivity in a TMD device.
Sasha Maslov
Then the group made the device superconduct just by tweaking the strength of the applied electric field. That result, which the researchers reported in Nature (opens a new tab) in October, doesn’t neatly fit any popular theory of superconductivity.
“It really smells like something else is going on,” Vishwanath said.
The Most Exotic Beast Yet
Even as superconductivity has spread to the TMDs, graphene continues to astonish. Over the summer, a graphene device produced a mythical form (opens a new tab) of superconductivity.
“It is phenomenologically different from all other superconductors,” said Long Ju (opens a new tab) of MIT, leader of the group that found it. And that’s “comparing it to any superconductor that has been discovered since Kamerlingh Onnes in 1911.”
Twisting is too messy for Ju’s tastes; the moiré patterns tend to get disrupted by wrinkles in the sheets that make every device a little different. Instead, he studies a staircase-like arrangement of four graphene layers that can also slow electrons down. The challenge is to spot which graphene flakes naturally have this staircase arrangement — something Ju accomplishes with the aid of an infrared camera. “You don’t need to pick up four different layers and stack them,” Ju said. “Nature does it for you. You just need to have the right pair of eyes to see them.”
Last year, Ju’s group made a splash when they placed a five-layer graphene flake on an insulator at a twisted angle and observed a rare electron behavior that normally requires a strong magnetic field to induce. Theorists questioned whether the twist was essential, so he and his team went back to see what would happen when they took the twist out. “We found something that was more bizarre,” Ju said.
As they changed the strength of the electric field that they applied to the material, they found several settings where resistance vanished. In two cases, the superconductivity flickered, with resistance coming and going. Strangely, when they switched on a nearby magnet, the flickering stopped. Magnets normally kill superconductivity, but here, they strengthened it. “This existed only in the imagination of theorists,” Ju said.
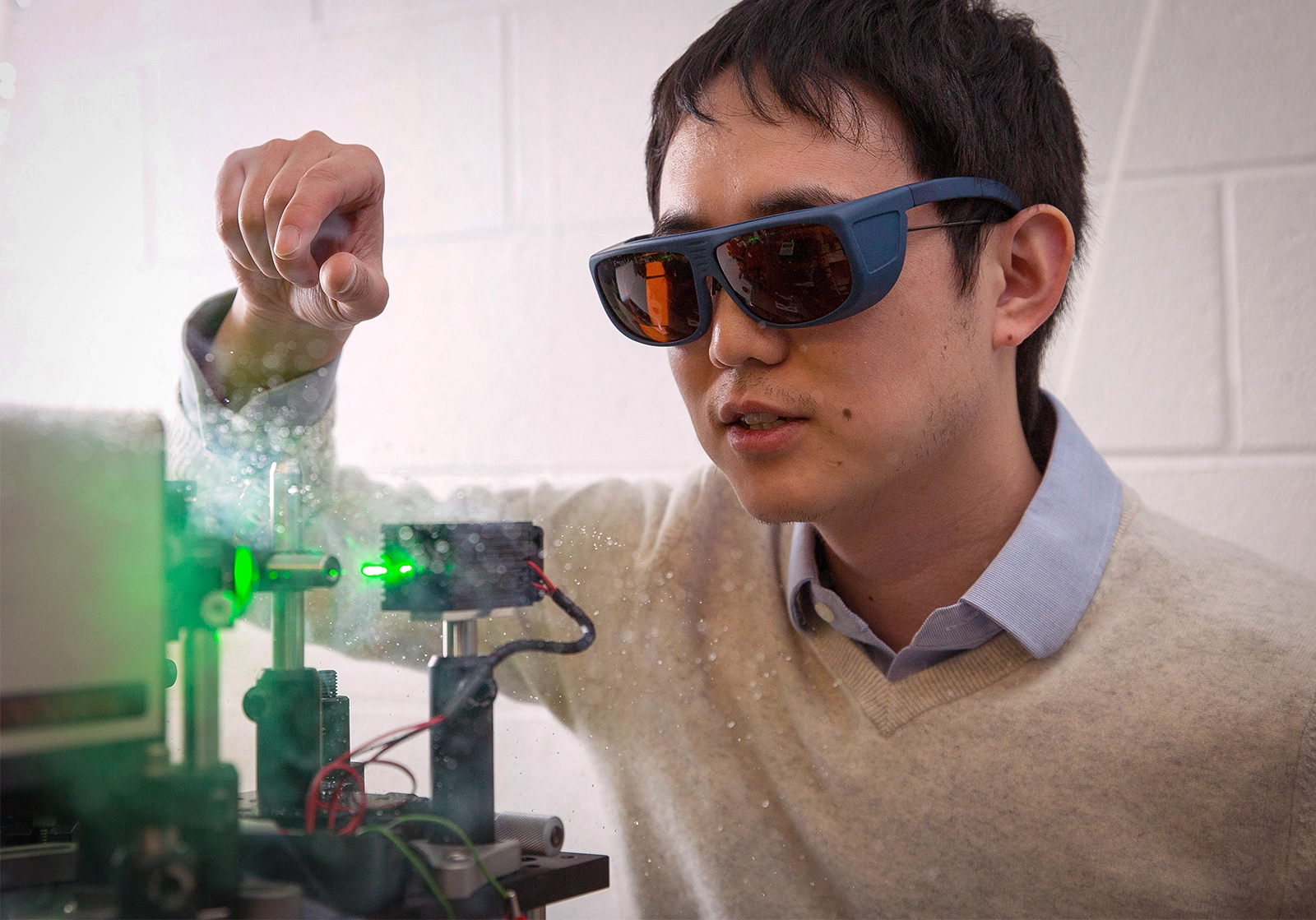
Long Ju helped discover a truly unprecedented superconductor — one that grows stronger in the presence of magnetism.
Courtesy of Long Ju
Ju’s group suspects that their graphene staircases are creating the conditions for electrons to pair up and rotate. But they think that in their graphene devices, all pairs tend to rotate in the same direction — either clockwise or counterclockwise — and flickers appear when pairs aren’t all rotating uniformly. The magnetic field stamps out the flickers by pushing any wayward pairs to align with the overall gyre. A material with such a preferred internal direction is called “chiral,” but chirality has long been thought to preclude superconductivity, since it distinguishes leftward- and rightward-moving electrons in a way that should stop pairs from forming.
“People thought you would not get superconductivity in this setting,” Vishwanath said. “It really calls for something entirely new.”
In fact, it’s so unusual that other researchers are waiting for more experiments to verify it. “It’s probably still an evolving story,” Mak said. “It just needs additional data to fully confirm whether it’s a chiral superconductor or not.”
Theorists, meanwhile, have published new theories of how chiral superconductivity might happen. Fu and collaborators proposed (opens a new tab) the following recipe in September: You start with electrons arranged to form a repeating crystal — like in an insulator, except in this case the electron grid is free to float independently of the background atomic nuclei. Then the electron grid relaxes, and its ripples pair electrons the way phonons do. Fu stressed that this is just one possibility, noting, “We’re in uncharted territory.”
A True Understanding
While physicists can’t say for sure what’s pairing electrons in these 2D materials, they feel more confident that there are multiple ways to do it. Electrons organize into all sorts of materials, from insulators to magnetic metals to electronic crystals, and slight disturbances seem poised to tip many of these materials into superconducting electron pairs.
Being able to directly see what happens when they add more electrons to a material or slightly weaken its electric field lets physicists quickly try out an unprecedented number of recipes and see which ones lead to superconductivity.
“The real promise,” Dean said, is that each of these devices is “a tunable lab in which we can make basically any other material.”
The experimentalists are amassing a treasure trove of data for theorists to explain. Mak and Shan hope that this abundance will let theorists predict ways to create superconductivity that experiments can confirm. That would demonstrate a true understanding of the phenomenon, which would mark both an academic achievement and a key step toward designing materials for revolutionary new technologies.
But for now, experimentalists are still the ones leading the way. “Everyone’s rushing as fast as they can,” Yankowitz said. “I can’t believe that we’re six years in and you can’t take a break.”