Physicists Spot Quantum Tornadoes Twirling in a ‘Supersolid’
Introduction
In a lab nestled between the jagged peaks of the Austrian Alps, rare earth metals vaporize and spew out of an oven at the speed of a fighter jet. Then a medley of lasers and magnetic pulses slow the gas nearly to a halt, making it colder than the depths of space. The roughly 50,000 atoms in the gas lose any sense of identity, merging into a single state. Finally, with a twist of the ambient magnetic field, tiny tornadoes swirl into existence, pirouetting in the darkness.
For three years, the physicist Francesca Ferlaino (opens a new tab) and her team at the University of Innsbruck worked to image these quantum-scale vortices in action. “Many people told me this would be impossible,” Ferlaino said during a tour of her lab this summer. “But I was so convinced that we would manage.”
Now, in a paper published today in Nature (opens a new tab), they’ve published snapshots of the vortices, confirming the long-sought hallmark of an exotic phase of matter known as a supersolid.
The supersolid, a paradoxical phase of matter that’s simultaneously the stiffest of solids and the flowiest of fluids, has fascinated condensed matter physicists since its prediction in 1957. Hints of the phase have been mounting, but the new experiment secures the last major piece of evidence for its existence. The authors believe the vortices that form in supersolids can help explain properties in a range of systems, from high-temperature superconductors to astronomical bodies.
The vortices might show how matter behaves in some of the most extreme conditions in the universe. Pulsars, which are spinning neutron stars — the extraordinarily dense corpses of burnt-out stars — are suspected to have supersolid interiors. “This is actually a really good analogue system” for neutron stars, said Vanessa Graber (opens a new tab), a physicist at Royal Holloway, University of London in the United Kingdom who specializes in these stars. “I’m really excited about that.”
Rigid and Runny
Imagine spinning a bucket filled with different kinds of matter. A solid will twirl along with the container because of the friction between the bucket and the material’s rigid lattice of atoms. A liquid, on the other hand, has less internal friction, so it will form a big vortex in the center of the bucket. (The exterior atoms rotate with the bucket while the inner ones lag behind.)
If you make certain liquids cold and sparse enough, their atoms begin interacting across longer distances, eventually linking together in one giant wave that flows perfectly without any friction. These so-called superfluids were first discovered in helium in 1937 by Russian (opens a new tab) and Canadian (opens a new tab) physicists.
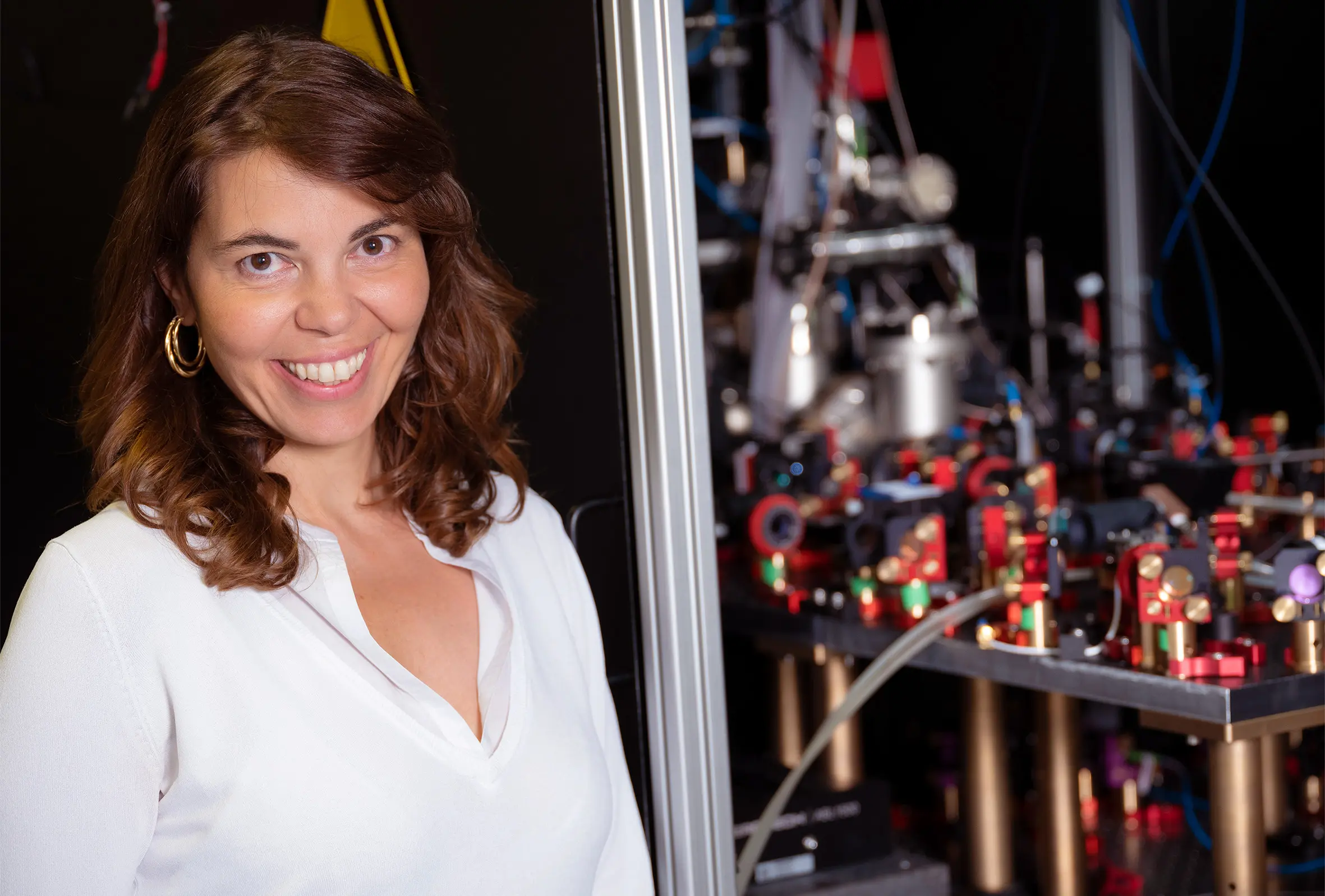
Francesca Ferlaino, a physicist at the University of Innsbruck, has observed the hallmark feature of supersolids.
M Vandory/University of Innsbruck
Try spinning a bucket of superfluid, and it will remain at rest even as the bucket rotates around it. The superfluid still rubs against the bucket, but the material is totally impervious to friction until the container reaches a certain rotational speed. At this point, resisting the urge to rotate, the superfluid suddenly spawns a single quantum vortex — a whorl of atoms surrounding a column of nothingness that extends to the bottom of the bucket. Continue to speed up the container, and more of these perfect tornadoes will slither in from the rim.
Twenty years after superfluids were discovered, the American physicist Eugene Gross suggested (opens a new tab) that the same quantum collectivism could emerge in solids. Physicists debated for decades (opens a new tab) whether this bizarre superfluid-solid hybrid could exist. Eventually, a theoretical picture emerged for the supersolid. By adjusting the magnetic field around a superfluid, you can reduce the repulsion between atoms in such a way that they begin to clump together. Those clumps will all align with the magnetic field but repel one another, self-organizing into a crystalline pattern while retaining their strange frictionless behavior.
Put a supersolid in a rotating bucket, and atoms will shift in sync such that the lattice of clumps will appear to revolve with the container, much like a solid. But, like a superfluid, when spinning fast enough the material will still break out into vortices, which will get pinned between the atom clumps. The supersolid will be at once rigid and runny.
Gross’ prediction launched a long hunt for supersolids in the lab.
Ferlaino Group
Researchers first announced a discovery in 2004, only to walk back their claim (opens a new tab). New bursts of activity (opens a new tab) came in 2017 (opens a new tab) and then again in 2019, when groups from Stuttgart (opens a new tab), Florence (opens a new tab) and Innsbruck (opens a new tab) found promising signals of supersolidity in one-dimensional systems. The groups started with gases of dysprosium and erbium atoms, which are intrinsically magnetic enough to act like little bar magnets. Applying a magnetic field triggered the atoms to naturally group together into regularly spaced clumps, forming a crystalline lattice. Then, when the researchers lowered the temperature and density, interactions between the atoms caused them to naturally oscillate as one coherent wave, complete with all the features of a superfluid.
The 2019 experiments caught a glimpse of the “two competing natures” of the supersolid, said Elena Poli, a graduate student on the Innsbruck team. Since then, the group has expanded their putative supersolid from one dimension to two (opens a new tab) and probed it for different predicted (opens a new tab) properties (opens a new tab).
But “what was missing was basically the smoking-gun evidence” of supersolids, said Jens Hertkorn (opens a new tab), a physicist at the Massachusetts Institute of Technology and a former member of the Stuttgart team. The hallmark of superfluidity is the array of vortices that spawns upon rotation. Despite years of trying, “nobody has spun a supersolid successfully before,” Hertkorn said.
Spinning a Supersolid
To observe how their supersolid responds to rotation, the Innsbruck crew used a magnetic field as a spoon to stir the internal magnetic fields of the atoms about 50 times a second. That’s fast enough to trigger vortices, but gentle enough to preserve the quantum phase. “It’s a very, very delicate state — any small change would destroy it,” Ferlaino said.
Spotting those little cyclones was a bigger challenge. The group spent three years quantum-storm chasing. Eventually, they executed a proposal (opens a new tab) from 2022 by Alessio Recati, a physicist at the University of Trento. He suggested forming vortices in the supersolid phase, then melting the material back into a superfluid in order to image the vortices with higher contrast.
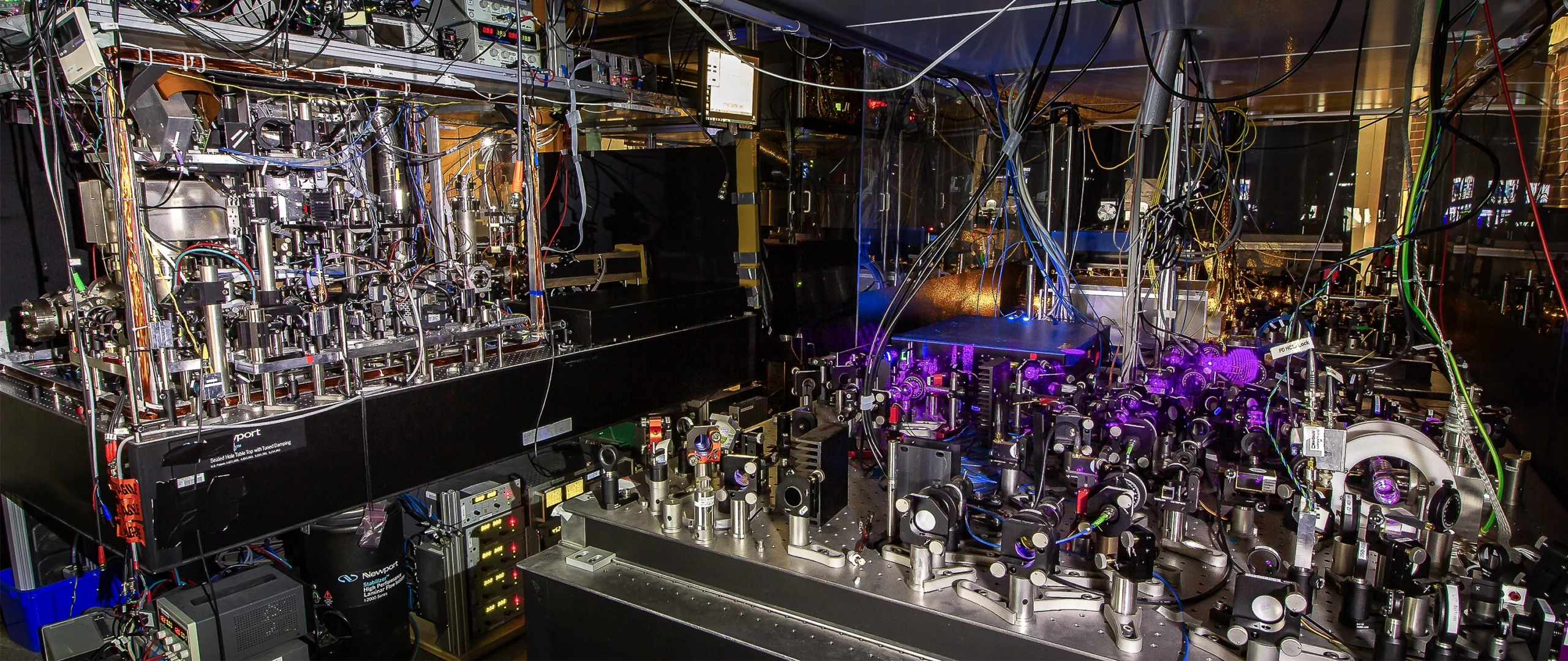
Francesca Ferlaino’s lab at the University of Innsbruck.
Patscheider
One Friday evening early last year, three grad students burst into a dim pub near the Innsbruck campus holding a laptop. They were looking for two of the team’s postdocs, who verified that they’d captured a tornado in their quantum gas. “It was exceptionally exciting,” said Thomas Bland, one of the postdocs. The grad students returned to the lab, and Bland and his colleague stayed for a celebratory round.
“We all believe that it is a quantum vortex,” said Recati, who was not involved with the experiment. He’s waiting for experimentalists to measure the rotational speed of the tornadoes to fully corroborate theoretical predictions, but the images alone are a satisfying validation, he said. “This is very relevant for the whole physics community.”
Hertkorn wants to see the results replicated by other groups and to track how the signals change over different experimental conditions. Still, he commends the Innsbruck team for their persistence in making such a challenging measurement. “It’s just experimentally really impressive that this is observable,” he said.
Cosmic Connections
This past May, Ezequiel Zubieta (opens a new tab) was lunching on stewed rolls in a small town outside Buenos Aires when he witnessed a dead star convulsing from his laptop screen. Zubieta, an astronomy grad student at the National University of La Plata, had been tracking the impressively stable rotation of the Vela pulsar, the magnetized remnant of a massive star that exploded roughly 11,000 years ago.
As it twirls, Vela shoots beams of radiation from its poles that flash on Earth 11 times per second, with a regularity that rivals the best clocks humans can build. But that day, the star spun around 2.4 billionths of a second faster than usual.
For decades, astronomers have wondered what could cause these massive objects to suddenly speed up their rotation. Many hope that these pulsar glitches can help them decipher the inner workings of these peculiar cosmic lighthouses.
Scientists know that stellar corpses are densely packed with neutrons — one teaspoon of neutron star material would weigh as much as Mount Everest. No one is sure what happens to neutrons in such conditions. But astronomers suspect that, in a layer below the star’s solid outer crust, pressurized neutrons form clumps that take on unusual shapes, which they often refer to as “nuclear pasta.” The leading models feature phases resembling gnocchi, spaghetti and lasagna.
At a conference in 2022, Ferlaino overheard some astronomers discussing the putative qualities of nuclear pasta. Many believe that the pastalike clumps of neutrons would merge to form a superfluid, but it’s unclear how that material could give rise to glitches. Ferlaino suspected that the glitches could be a sign of the supersolids she’d been cooking up in her own lab, so she decided to investigate.
The pressurized neutrons that fill neutron stars are thought to take on an array of possible shapes known as “nuclear pasta.”
Ferlaino Group
Last year, her team used a computer simulation of their supersolid to model what would happen if a similar material existed inside a spinning neutron star. They found that after vortices form, one of them can get dislodged and bump into its neighbor, setting off a tornado and avalanche that transfers its energy to the container. Enough of these tornado collisions could briefly speed up the neutron star’s rotation, resulting in a glitch (opens a new tab), they proposed.
Graber, who had published a review of laboratory analogues for neutron stars (opens a new tab) several years earlier, was thrilled to come across the paper. “Oh my God, there is something else out there that I can use,” she recalled thinking about the various properties of rotating supersolids described in the paper. “Just reading through the text, I was like, ‘This is what I have, and this is what I have, and this is what I have.’”
Now that Ferlaino’s group has identified vortices in their supersolid, they plan to investigate how the tornadoes form, migrate and dissipate. They also want to replicate the putative mechanism for pulsar glitches, to show how an avalanche of vortices might prompt a real-world supersolid to speed up its spin. Physicists also hope to use these studies to decipher other exotic phases of matter where vortices are expected to play a key role, such as in high-temperature superconductors (opens a new tab).
Meanwhile, astronomers like Graber and Zubieta hope this work will enable a new diagnostic tool for pulsars. With a better understanding of vortex dynamics, they may be able to use pulsar glitch observations to infer the composition and behavior of nuclear pasta.
“If you can understand how that physics works on a small scale, that’s really valuable for us,” Graber said. “I can’t use a telescope and look inside a neutron star’s crust, but they essentially have that handle.”
Ferlaino, whose group is on the lookout for other systems that may sport supersolidity, sees the applications as a reflection of the fundamental connectedness of nature. “Physics is universal,” she said, and “we’re learning the rules of the game.”